Biofilm Challenges in Dairy
Abstract
Milk collected from the udder of healthy cows is virtually free of contaminating bacteria but microbial contaminants are introduced during milking, storage, processing, and distribution. Cleaning and sanitation failures allow contaminating organisms to accumulate in dairy processing equipment, potentially leading to the development of biofilms that can become a significant and persistent source of contamination of dairy products impacting product quality and safety. Biofilms are aggregations of bacterial cells that form on surfaces of equipment or environmental fomites. Bacteria making up biofilms are interconnected by extracellular polymeric substances that accelerate growth and protect the cells from heat and chemical sanitizers. Biofilms represent a critical challenge to the dairy industry where they act as the principal reservoir of microbial contaminants. Good manufacturing practices that include good hygienic practices and Hazard Analysis and Critical Control Point (HACCP) quality management systems implemented both at the farm and processing plant level are vital to protect the quality and safety of the dairy supply.
Introduction
Biofilms are responsible for or contribute to chronic
illnesses and nosocomial infections; contaminate medical devices and equipment; food and dairy contamination and spoilage; and even foul the hulls of ocean-going ships. At the same time, biofilms have many important industrial uses, primarily associated with treating water and wastewater to remove biological pollutants or heavy metals and aid oil recovery from wells.4,9 In the dairy industry, the presence of biofilms is most often — although not always — cause for concern.
The formation of biofilms on the surfaces of dairy production or processing equipment is a critical factor that can lead to a severe and persistent contamination of dairy products and constitutes a significant challenge to environmental and equipment hygiene. Bacterial adhesion to stainless steel and other materials found in dairies is a complex biological process that is influenced by the physiology of the organisms, the physical chemistry properties of the surface, and the environmental conditions, such as pH, temperature, and nutrient availability.4,13
Biofilms are immobile (or sessile) aggregations of microcolonies of microorganisms attached to surfaces and living at an interface between the surface and a liquid phase. Biofilms are embedded in and protected by an organic polymer matrix consisting of extracellular polymeric substances (EPS) that are formed and excreted by the bacteria comprising the biofilm. This EPS matrix is composed of long-chained carbohydrate molecules (polysaccharides), proteins, extracellular DNA (eDNA), and lipids (lipopeptides).2,7,10 It is estimated that 40-80% of all bacterial species on earth are capable of forming biofilms, with up to 90% of microbial populations found in nature existing as biofilms rather than discrete organisms floating freely in the environment (called planktonic cells).2,4
Biofilm Formation
Biofilms form in a predictable but complex fashion, beginning when planktonic cells adhere to a surface in a weak, reversible, and non-specific manner and begin to excrete EPS.4 This is the first in a series of five main phases of biofilm formation that include: (i) the reversible attachment phase; (ii) the irreversible attachment phase, where interaction between surfaces and lipopolysaccharides found on hair-like bacterial appendages (pili or fimbriae) form tight ionic attachments; (iii) production of EPS by the cells forming the biofilm; (iv) biofilm maturation during which cells grow, cell density increases, and cells synthesize and release signaling molecules allowing them to sense and communicate with each other; and (v) dispersal or detachment phase, where the cells depart in large numbers to become planktonic cells again.10
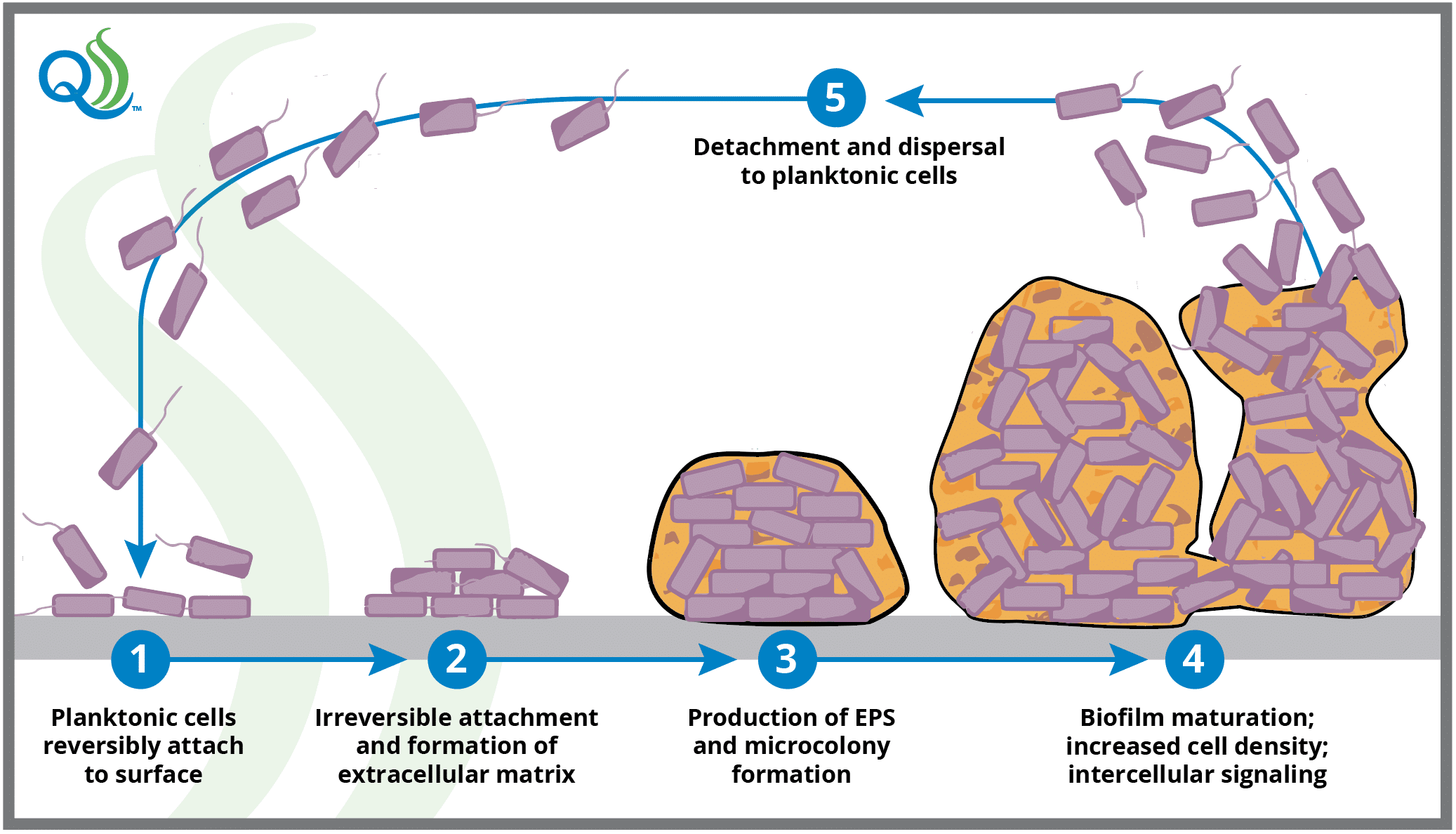
Once established, biofilm maturation typically occurs within 72 to 144 hours, although maturation may take up to 240 hours for some bacterial species.6 Maturity occurs by increasing the bacterial population density and deposition of the EPS matrix. Although most cellular detachment occurs following biofilm maturation (in the detachment phase), cells may detach during each stage of biofilm development, making biofilms a potential and persistent contamination risk immediately upon initiation.
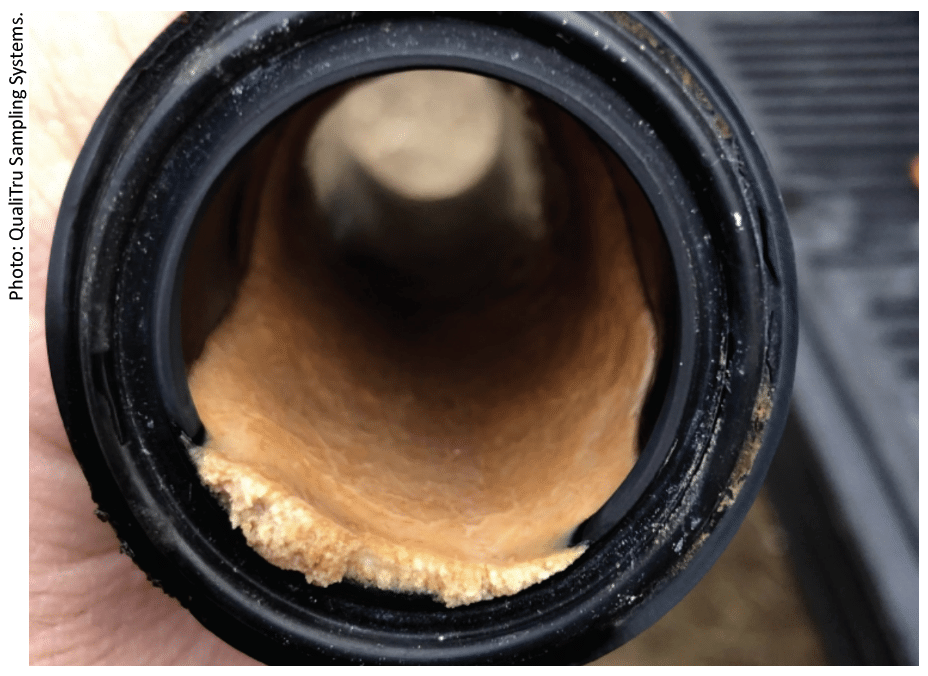
Importance of Biofilms in the Dairy Industry
Biofilms can form on various materials and surfaces in dairy farms or processing plants. Teflon, rubber, stainless steel, glass, polypropylene, ceramic or other tiles, and most other materials found in dairy production or processing environments will support biofilm formation. As such, biofilms may become established on environmental fomites, such as walls, floors, and drains; in milking parlors or equipment; inline milk filters; surfaces of stainless steel equipment, such as milk storage tanks, pasteurizers, and piping; gaskets; valves; tools; milk handling devices; and more.1,2,4,7,9 The widespread nature of biofilm-forming microbes in the dairy industry represents an important source of bacterial contamination affecting raw and pasteurized liquid and dry dairy products. This persistent contamination may result in foodborne disease, food spoilage, and economic loss.
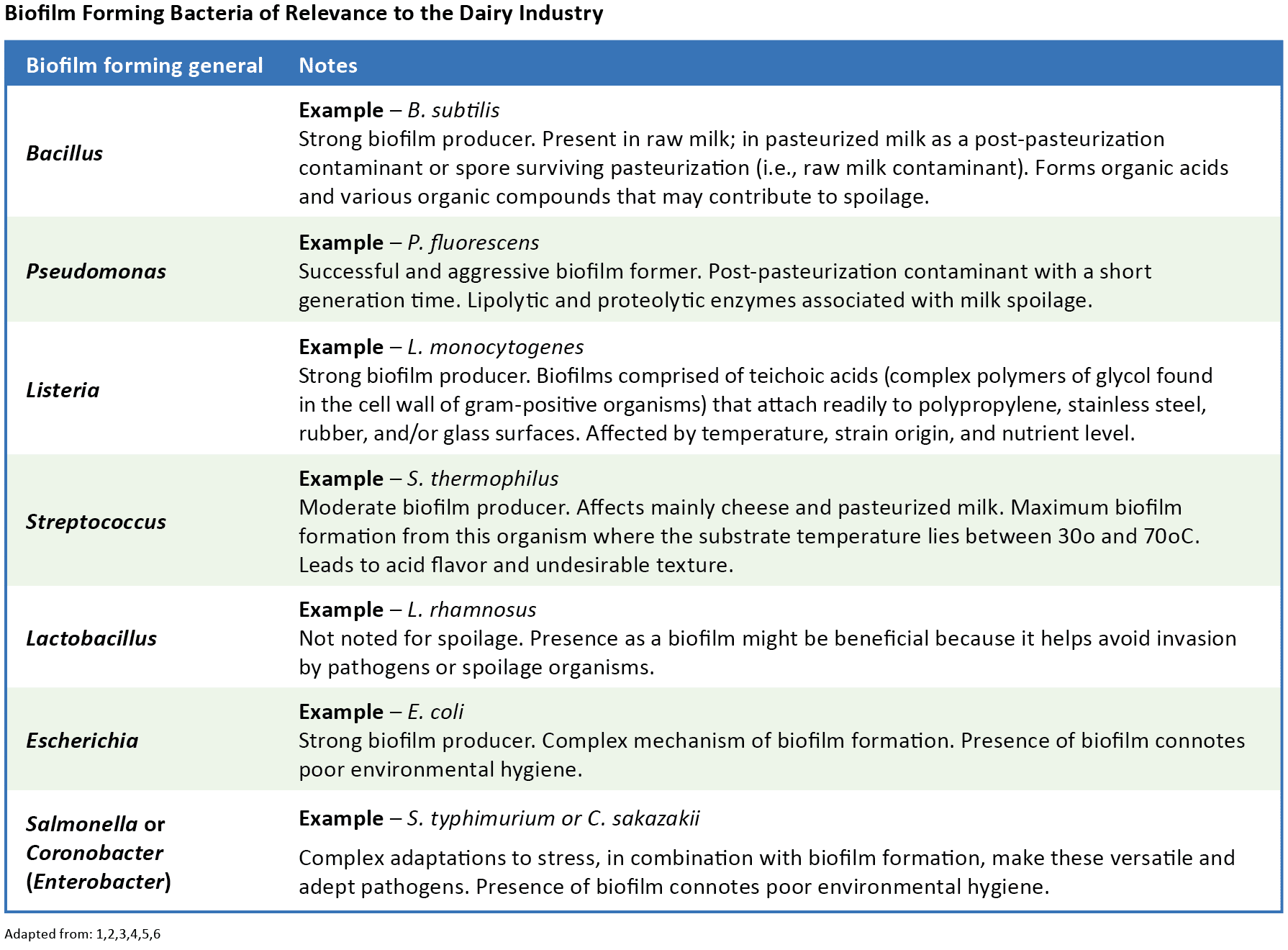
Biofilm Formation on Dairy Equipment
The strength of biofilm adhesion to the surface of dairy equipment is influenced by the hydrophobic properties of the surface of the organisms, the presence of their pili (or fimbriae), and the physiochemical properties of the surface.12,13 Initial, reversible adhesion is due to Van der Waals forces, weak, non-ionic molecular attractions between the organism and the surface. Irreversible surface bonding develops as attachments become ionic. Most bacteria have negatively charged surfaces with components that form strong hydrophobic interactions with surfaces. The presence of polysaccharides or proteins on the surface of the equipment (as may be found if improperly cleaned) helps bacteria adhere to the surface and contributes to the development of complex biofilms containing two or more species of organisms.4
Because microorganisms can survive on surfaces of dairy equipment, hygienic requirements such as are adopted to apply to surfaces of dairy equipment covering such concerns as steel grade, relief, and surface roughness. Even polished stainless steel, however, may have surface roughness deformities ranging from <0.5 to 1.0 microns, with reports as high as 3.2 microns. Biofilms can form on a stainless steel surface with roughness as low as 0.16 microns, although formation is slow, and the biofilm may not develop to full maturity or high density. At a surface roughness of 0.63 microns, spherical-shaped organisms (e.g., Staphylococcus sp.) will form biofilms approximately 1.4 times faster than rod-shaped organisms, such as Pseudomonas sp. or Bacillus sp. This is because the spherical shape of cocci can fit into the hollows more easily than rod-shaped organisms that must adhere to the surface over their entire length.11
In a 2019 study, Stadnyk, et al.11 showed that at 0.95-micron roughness, both cocci and rod-shaped organisms complete the first phase of biofilm formation within three hours in laboratory incubation conditions, and with a roughness of 2.687 microns, both formed fully established biofilms within 3-24 hours. This research shows that bacteria can attach freely in the hollows found on stainless steel surfaces to begin the first stage of biofilm formation. The formation speed increases as roughness exceeds 0.8 microns. It also shows that polishing surfaces to a roughness of <0.5 microns represents the best method for controlling biofilm formation.
Early biofilm attachment is weak, and the contaminants can be easily removed through proper sanitation procedures utilizing high-temperature cleaning solutions to remove fat and protein residue that can harbor bacteria and provide attachment for biofilm formation. Once biofilms form and mature, they are harder to remove and require more aggressive action to eliminate. Standard cleaning-in-place procedures may not be sufficient to eliminate the contamination once established.1,4,5,9 Bacteria embedded in a biofilm matrix are protected and become more resistant to heat, cleaning, and sanitizing chemicals than their corresponding planktonic cells. Aggressive cleaning procedures may be required to eliminate an established biofilm. Methods for evaluating and monitoring the cleaning results of a dairy plant or farm milk production operation should be built into a HACCP program. They should include programs for evaluating processing areas for biofilm sanitation.
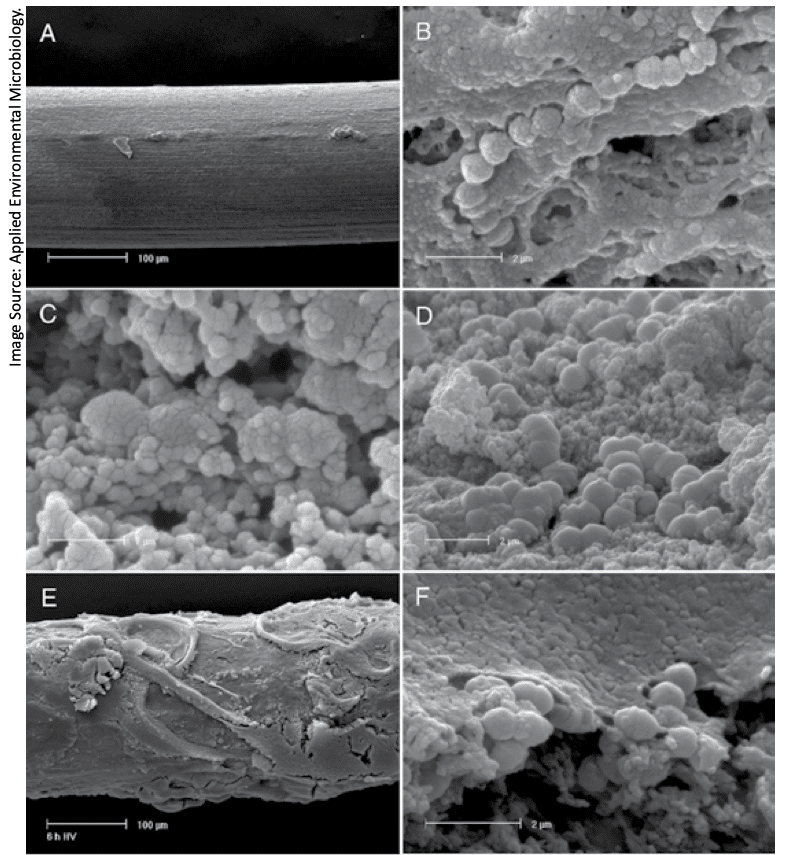
Detecting Biofilms
Several methods exist for the detection of biofilms, but only some have practical applications for use in the dairy industry. Techniques such as light microscopy, electron microscopy, and confocal laser scanning microscopy are useful in directly observing biofilms in research. Mass spectrometry (MS) can be used to characterize EPS swabbed from surfaces, and matrix-assisted laser desorption ionization (MALDI) coupled with time-of-flight MS (TOF) is often used in dairy research to identify specific bacteria. These are research techniques, however, that are not suitable for routine application.
Detecting biofilms in a milk production or processing plant environment is difficult, but several techniques exist. Examples of methods to detect potential sources of biofilm contamination include:2,5,8
- Direct observation. After cleaning, protein residues on stainless steel surfaces become potential biofilm attachment sites. These can often be detected by use of wave-alternating UV light, such as BactiscanTM, or may be seen as a “rainbow” appearance on the surface of stainless steel milk contact surfaces, such as tank walls. The presence of protein residue on the surface is no assurance of the presence of biofilm, but it may suggest inadequate cleaning, which increases the probability of biofilm formation. Often, clean-appearing equipment surfaces will feel slimy, also indicating biofilm growth.
- ATP bioluminescence. Adenosine Triphosphate (ATP) bioluminescence is a method by which ATP in bacteria reacts with the enzyme luciferase to emit light. A luminometer measures the light and quantifies the contamination on the test surface based on the amount of light emitted. Unfortunately, this method is unreliable for biofilm detection because cells embedded in a biofilm matrix use less energy and produce less ATP than their mobile planktonic counterparts. As such, the bioluminescence test may provide a negative result even if a biofilm is present.
- Hyperspectral fluorescence imaging. Studies conducted by the Food and Drug Administration (FDA) in 2008 determined that portable hyperspectral fluorescence imaging devices could be used to inspect food contact surfaces for biofilm contamination. Since then, several such devices have come to market but remain very expensive.
- BioFinder and Realzyme biofilm detection. BioFinder and Realzyme are proprietary products sprayed on food and dairy contact surfaces to detect the presence of biofilms. The chemicals in these products react with the bacterial enzyme catalase to create white bubbles that contrast with the color of the product. False positives are possible if the food material has not been sufficiently cleaned from the surface and contains the catalase enzyme.
All these direct observation methods are limited by the need for open and easily observable surfaces. None of these methods are suitable for finding biofilms in closed equipment environments, such as milk lines, heat exchangers, valves, most milk storage tanks, and other such equipment.
The only sure way to find biofilm contaminants in a complex milk production or processing environment is to isolate contamination hotspots, which are locations within a process where sporadic bacterial sloughing leads to spikes in microbiological load.5 Microbiological tests to identify these hotspots may be as generic as total plate or coliform counts or as specific as tests for environmental or contagious pathogens, spore-forming organisms, or gram-negative psychrotrophic spoilage organisms. This hotspot isolation method should be used to monitor process contamination hazards. For example, microbiological testing of aseptic samples collected upstream and downstream of equipment identified as a potential contamination hazard will isolate bacterial contamination originating from the equipment. Equipment that may constitute a contamination hazard may include heat exchangers, inline filters, milk storage or blending tanks, valves and valve clusters, filling machines, evaporators, and others.
Unfortunately, if biofilms are not detected early enough, the result may be sporadic but persistent contamination that will decrease shelf life, introduce pathogens to finished products, or cause economic harm. Worse, if shelf life has already begun to fail due to higher-than-normal spoilage organism contamination, the possibility of biofilm formation must be considered, and strong measures must be taken to eliminate it.
Conclusion
Biofilm formations represent a profound challenge to the dairy industry where they act as the principal reservoir of microbial contamination. This persistent threat of contamination by spoilage and pathogenic organisms thriving in biofilms has the potential to impact product quality and safety that may lead to economic loss. Measures to control the introduction of contaminating microbes into dairy products and safeguarding against the development of biofilms on production or processing equipment are critical to protecting the food supply. Vigilance is needed to detect and isolate biofilms in dairy production and processing environments and find ways to eliminate them once formed. It is emphasized that good manufacturing practices, including good hygienic practices and Hazard Analysis and Critical Control Point quality management systems implemented both at the farm and processing plant level are vital to protect the quality and safety of the dairy supply.
References 1. Austin JW, G Bergeron. Development of bacterial biofilms in dairy processing lines. J Dairy Res. 1995; 62:509-519. 2. Bhosale S, P Brahmane, A Kubade, RJ Desale. Biofilm in the dairy industry: Detection and common process for control biofilms. Pharma Innovation J. 2021; SP-10(8):809-817. 3. Brown S, JP Santa Maria Jr, S Walker. Wall Teichoic Acids of Gram-Pos- itive Bacteria. Annu Rev Microbiol. 2013; 67: doi:10.1146/annurev-mi- cro-092412-155620. 4. Cherif-Antar A, B Moussa-Boudjemaa, N Didouh, K Medjahdi, B Mayo, A Belen Florez. Diversity and biofilm-forming capability of bacteria recovered from stain- less steel pipes of a milk processing plant. Dairy Sci Technol. 2016; 96:27-38. 5. Cramer M. Biofilms: Impact on the Food Industry. Food Safety Mag. June/July 2012; Biofilms: Impact on the Food Industry | Food Safety (food-safety.com) 6. de Oliveira MMM, DF Brugnera, E Alves, R Hisldorf Piccoli. Biofilm formation by Listeria monocytogenes on stainless steel surface and biotransfer potential. Braz J Microbiol. 2010; 41:97-106. 7. Di Martino P. Extracellular polymeric substances, a key element in understand- ing biofilm phenotype. AIMS Microbiol. 2018; 4(2):274-288. 8. Hassan A, J Usman, F Kallem, M Omair, A Khalid, M Iqbal. Evaluation of differ- ent detection methods of biofilm formation in clinical isolates. Braz J Infect Dis. 2011; 15(4):305-311. 9. Latorre AA, JS Van Kessel, JS Karns, MJ Zurakowski, AK Pradhan, KJ Boor, BM Jayarao, BA Houser, CS Daugherty, YH Schukken. Biofilm in milking equipment on a dairy farm as a potential source of bulk tank milk contamination with Listeria monocytogenes. J Dairy Sci. 2010; 93(6):2792-2802. 10. Muhammad MH, AL Idris, X Fan, Y Gao, Y Yu, X Jin, J Qiu, X Guan, T Huang. Beyond Risk: Bacterial Biofilms and Their Regulating Approaches. Front Microbiol. 2020; 11(article 928):1-20. 11. Stadnyk I, T Hushtan, G Sabadosh, Y Yevchuk. Formation of microbial biofilms on stainless steel with different surface roughness. Potrav Slovak J Food Sci. 2019; 13(1)915-924. 12. Telford JL, MA Barocchi, I Margarit, R Rappuoli, G Grandi. Pili in Gram-positive pathogens. Nature Rev Microbiol. 2006; 4:509-519. 13. Yi K, AW Rasmussen, SK Gudlavalleti, DS Stephens, I Stojiljkovic. Biofilm For- mation by Neisseria meningitidis. Infection Immun. 2004; 72(10):6132-6138. 14. Bassi, D., Cappa, F., Gazzola, S., Orrù, L., and Cocconcelli, P. S. (2017). Biofilm Formation on stainless steel by Streptococcus Thermophilus UC8547 in milk environments is mediated by the proteinase prts. Appl Environ Microbiol., 83(8). https://doi.org/10.1128/aem.02840-16
Who is QualiTru?
Launched in 1983 by Darrell Bigalke, QualiTru Sampling Systems (formerly QMI) started with a single product (now called the QualiTru TruStream Septum). Designed for samples to be drawn a single time from each sterilized channel, the high-quality, food-grade quality septum dramatically reduces the risk of sample contamination.
In 1985, when serious outbreaks of dairy-related foodborne illnesses caused by Salmonella and Listeria sickened thousands of people in the U.S., Bigalke played a central role in helping shape the establishment and adoption of the new sampling standards in the dairy industry. Bigalke, the dairy industry, and key regulatory bodies all shared the same goal: to initiate process monitoring measures to reduce the chances of future foodborne illness outbreaks in the dairy industry.
Today, QualiTru Sampling Systems offers a full line of advanced, cost-effective, and easy-to-use systems that enable the dairy and liquid foods industries to perform inline aseptic process sampling to help ensure that their microbial testing is based on accurate sampling. QualiTru Sampling Systems has an unparalleled network of subject matter experts (SMEs) in the dairy and liquid food industries, enabling the company to provide quality in-house expertise for customer training and consultations.
From one man’s singular vision and commitment to apply science to stop foodborne illness tragedies, QualiTru Sampling Systems has grown into a worldwide company trusted to provide accurate and representative samples to the dairy and liquid food industries, helping them meet their needs for product quality and safety, public health, and business goals.
As we like to say at QualiTru:
Your Test Result Is Only as Accurate as Your Sample!
Why Partner with QualiTru?
QualiTru understands the liquid sampling challenges of our customers. QualiTru sampling systems provide fast, simple, safe, and accurate samples for bacterial and chemical quality analysis. Our systems help identify in-process microbial risks from issues such as biofilms, cracks, pinholes, and other potential contaminants, helping to ensure optimal sampling throughout your production process while reinforcing the safety and quality of your products.
Our unique four-part configurations can be customized to perform representative sampling, whether inline from the milking parlor to truck transport, from raw and pasteurized storage silos, and throughout commercial dairy and liquid food processing operations. This allows these industries to have accurate microbiological and chemical data from beginning to end, building transparency and confidence in product quality.
QualiTru’s sampling solutions have proven themselves for over four decades, with installations across 30+ countries. Our products comply with the 3‐A Standard 63‐04 (Sanitary Fittings) and are authorized to display the industry standard 3-A symbol.
QualiTru Sampling Systems’ regulatory approvals include:
- FDA M‐I‐06‐12 Needle Only Method
- FDA M‐I‐08‐5 Milk Tank Trucks
- FDA M‐I‐06‐6 Direct Load
- EC1935 compliant
- FDA M‐I‐12‐4 Universal Dairy Farm Samples
- FDA M-I-16-17 Appendix N Samples
In addition, as part of QualiTru’s focus on continuous improvement for exceptional customer satisfaction, we are proud to have earned ISO 9001:2015 certification for our services for the liquid food industry.

Ask Our Experts in Liquid Sampling Systems
On the heels of two dairy-related tragedies occurring in rapid succession in the 1980s, those four simple words inspired QualiTru’s founder to ask, “How can I help reduce the chances of future contaminations or bacterial outbreaks from ever claiming another life?”
So, “How can we help?” Please email us at [email protected] or click HERE to fill out a short form to be contacted by one of our experts.
Stay in Touch with QualiTru
- Bookmark our website to access educational resources quickly.
- Sign up for our monthly newsletter to receive blog posts and resources.
- Call 651.501.2337 to talk to a QualiTru representative.
- Email us for a quote or information at [email protected].
- Follow us on:
QualiTru Blog Library
Visit our website to browse our library of blogs authored by dairy, brewery and liquid food industry experts supporting our issues-to-solutions sampling approach. Popular blog posts include: